From Earth is a Nuclear Planet by Mike Conley (Author), Tim Maloney Ph. D. (Author), Stephen A. Boyd Ph. D. (Scientific Editor)
Author: THE KERNEL
Listen to chapter 2 as an audiobook here!
(Heads up: This chapter is a deep dive into the basics of radiation. You can skip it, skim it, or refer to it later, but reading it now will serve you well in the chapters ahead.)
Be not afraid. Unless you were born and raised in a salt mine, you’ve been bombarded with radiation your entire life. Welcome to planet Earth. Cosmic rays are but one small example. These are mostly high-velocity protons from our sun, along with some high-energy gamma rays that come zooming down to us from beyond the solar system. Most swerve away upon entering the magnetosphere, but some still reach the planet surface. And if you’re in the way, they’ll zoom right through your body. [1]
About 13% of the background radiation we encounter can be attributed to cosmic rays, and the less atmosphere there is above us the more intense the effect. People living at high elevations, people at cruising altitude, and astronauts get zapped by zoomies worse than anyone. No one is left untouched.
(Note: Just to be clear, “zoomie” is US Navy submariner slang for radiation, and does not refer to dogs spinning in circles or running crazy around the house.)
We’ll be focusing on the zoomies that originate right here on Earth. Categorized as alpha, beta, or gamma radiation, they come from inside an atom’s nucleus. That’s why zoomies are called subatomic particles—they’re much smaller than any atom.
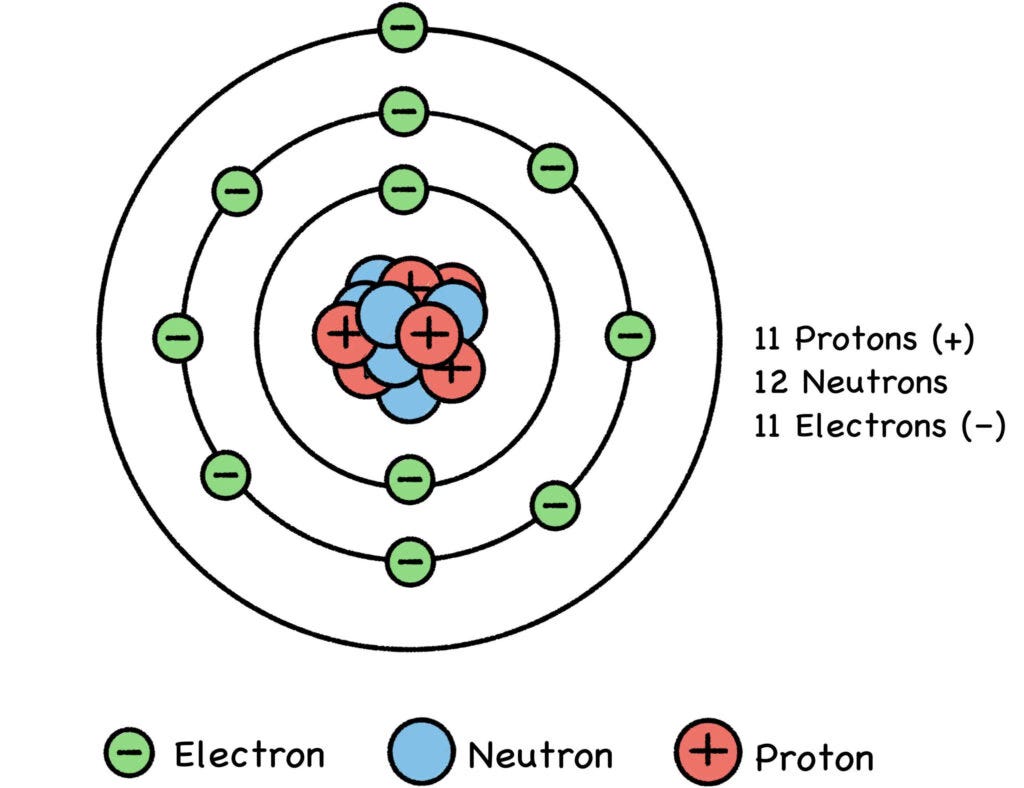
Fig. 4: The Sodium Atom
(Note: To keep our sketches simple, we don’t depict every proton and neutron. Just pretend the rest of them are on the far side of the nucleus.)
If you really want to understand the actual hazards of radiation, and the lack thereof, it’s important to get this zoomie stuff right, so put your thinking cap on and embrace your inner nerd. We’ll walk you through it.
The enormous nuclei (centers) of uranium and thorium atoms are two examples of zoomie emitters. These heavy metal elements, and the isotopes into which they decay, are found all over the world in rock, soil, and water. Only slightly radioactive, these atoms will throw off the occasional zoomie as they slowly transmute (change) into completely different elements, getting smaller and weaker over time. One of these elements is radium, which decays into the radon lurking in your basement (see below), and in morning dew. [2]
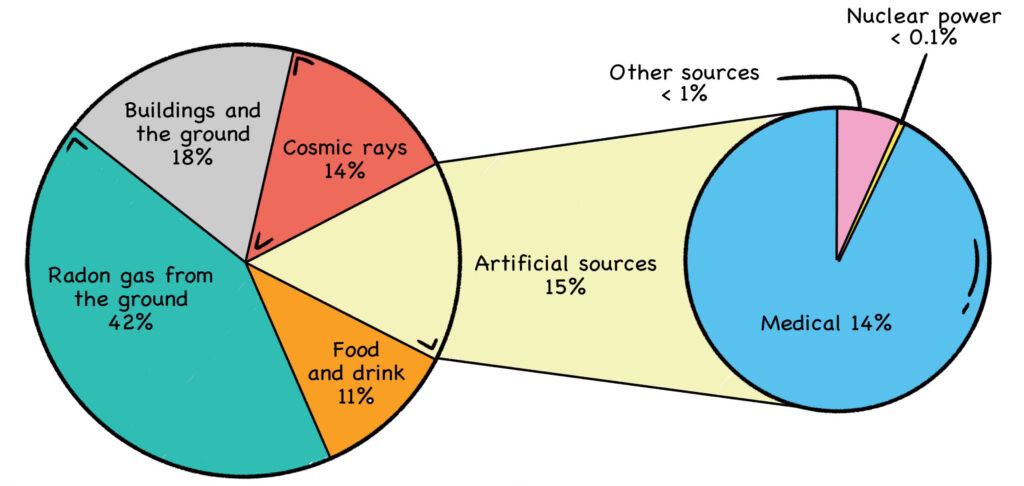
Fig. 5: Background Radiation
Establishing sensible safety standards requires a thorough understanding of the effects of radiation on the human body. But just as important, it also requires that we update our understanding—and our regulations—as science and medicine evolve.
Zoomies are everywhere, and there is no escape. They’re in sunlight; they’re even in bananas, loaded with nutritious potassium. Most potassium (K-39) is radiologically inert. But a tiny percentage (0.012%) of any batch of potassium is radioactive K-40. This is why the average banana contains about 0.1 microSievert of radiation.
As a matter of fact, bananas are so radioactive that a truckload can set off alarms at a border crossing; so can avocados. The average avocado has a bit less K-40, at about 0.08 microSievert. [3] The Department of Homeland Security (DHS) actually had to recalibrate their testing apparatus for incoming shipments.
“Banana Equivalent Dose” (BED) is a jocular way of assessing low doses of radiation, by comparing the doses we commonly receive in our daily lives with the consumption of ridiculous amounts of bananas. The point being is that we all need to dial back on our nuclear fears and keep low-dose radiation in perspective. While high doses can indeed be dangerous, low doses are not the dire threat that some claim them to be.
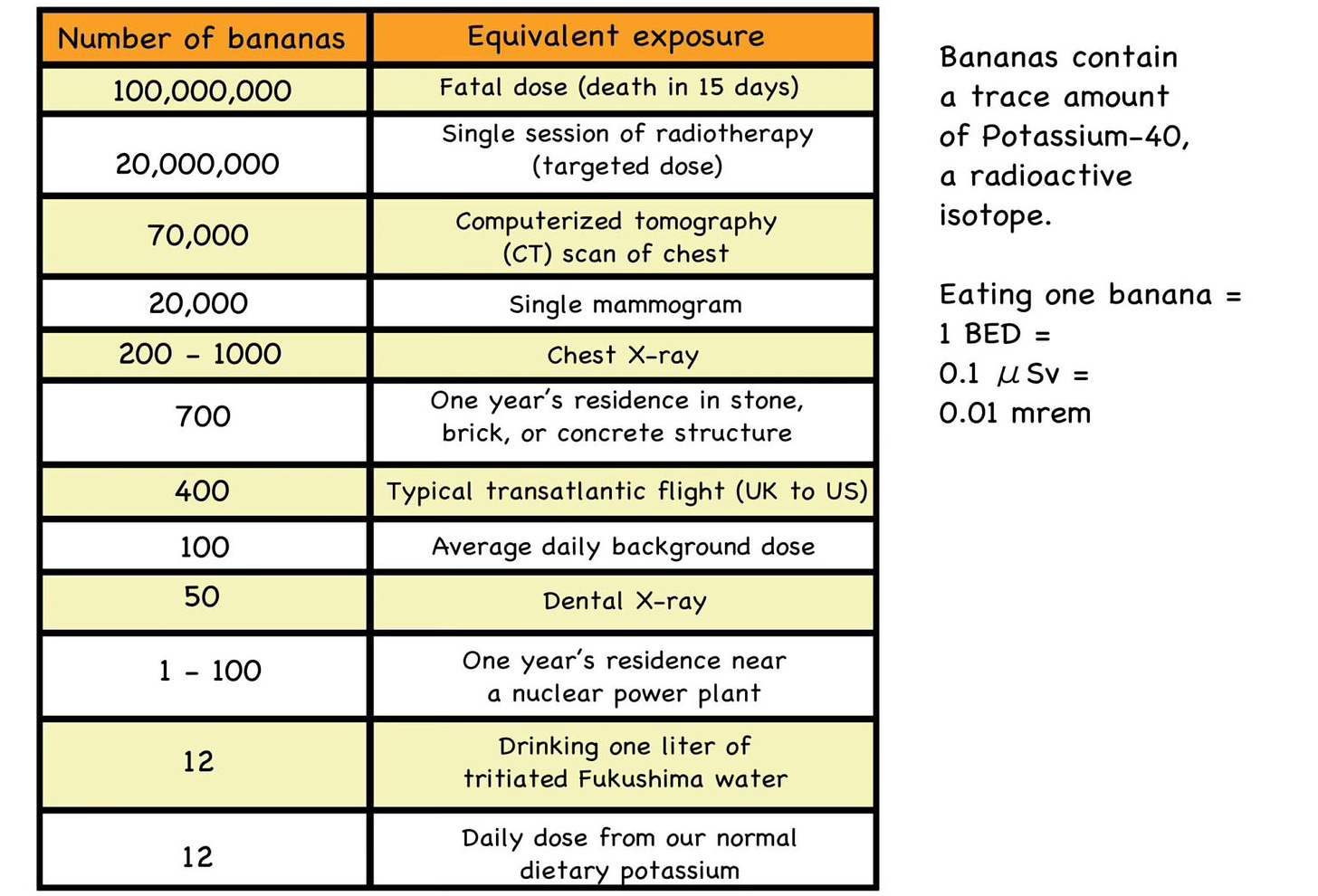
Fig. 6: Banana Equivalency Table
Source: https://livinglfs.org/mutants-vs-radiation-trying-to-understand-radiation-in-lfs/
NERD NOTE:
- Sievert (Sv) is the standard unit for quantifying the biological effect of a dose of ionizing radiation on a lifeform, called the effective dose.
- milliSievert (mSv) = one thousandth of a Sievert.
- microSievert (μSv) = one millionth of a Sievert.
We get even more zoomies from man-made sources like X-rays and CT scans. Zoomies are also in beer, and every time you down a frosty brew they zap you from within. (It’s from the potassium in the grains.) And then there’s all that guacamole.
Any granite has traces of radioactive material—this includes your kitchen countertop. Even clean seawater has a “becquerel count” of about 12,000 Bqs per cubic meter. Most of this comes from potassium-40 and rubidium-87, along with a smattering of uranium. To put this in perspective, adult humans have a continuous internal becquerel count of about 5,000, nearly all of it from the potassium in their cells—about 80% in muscle cells, and 20% in bone cells. [4] (See Chapter 15.)
NERD NOTE: A becquerel (Bq) is a standard measurement of basic radioactivity. One Bq simply means one decay emission per second, regardless of what is being radiated or how energetic the emission happens to be. Whenever an atom spits out a zoomie, that’s one becquerel.
Whatever subatomic particles an atom happens to emit—protons, neutrons, electrons, or gamma rays—the atom is classified as radioactive because it’s actively radiating zoomies. Even if it only ejects one weak zoomie every hundred years, it’s still considered radioactive.
A popular bogeyman, radon-222 contributes to nearly half the average background radiation we humans typically receive on this nuclear planet. The popular linear no threshold (LNT) model, which claims there is no safe dose of radiation, is flatly contradicted by the real-world data on radon.
The graph below summarizes a 1995 study of 1,729 US counties, comprising 90% of the American population at that time. [5] Increasing radon levels are shown on the x-axis (horizontal), and increasing cancer rates are shown on the y-axis (vertical). When the data is examined, it’s clear that the low-dose radon levels typically found in our homes do not correlate with increased rates of cancer. In fact, quite the opposite: Lung cancer mortalities are actually lower in households with higher-than-average radon levels.
In certain places, such as underground mines, radon can be much higher than average, and if it poses a risk it should of course be mitigated. But just like the mitigation of any potentially hazardous substance, the question is: To what degree? Science, not science fiction, should be our guide.
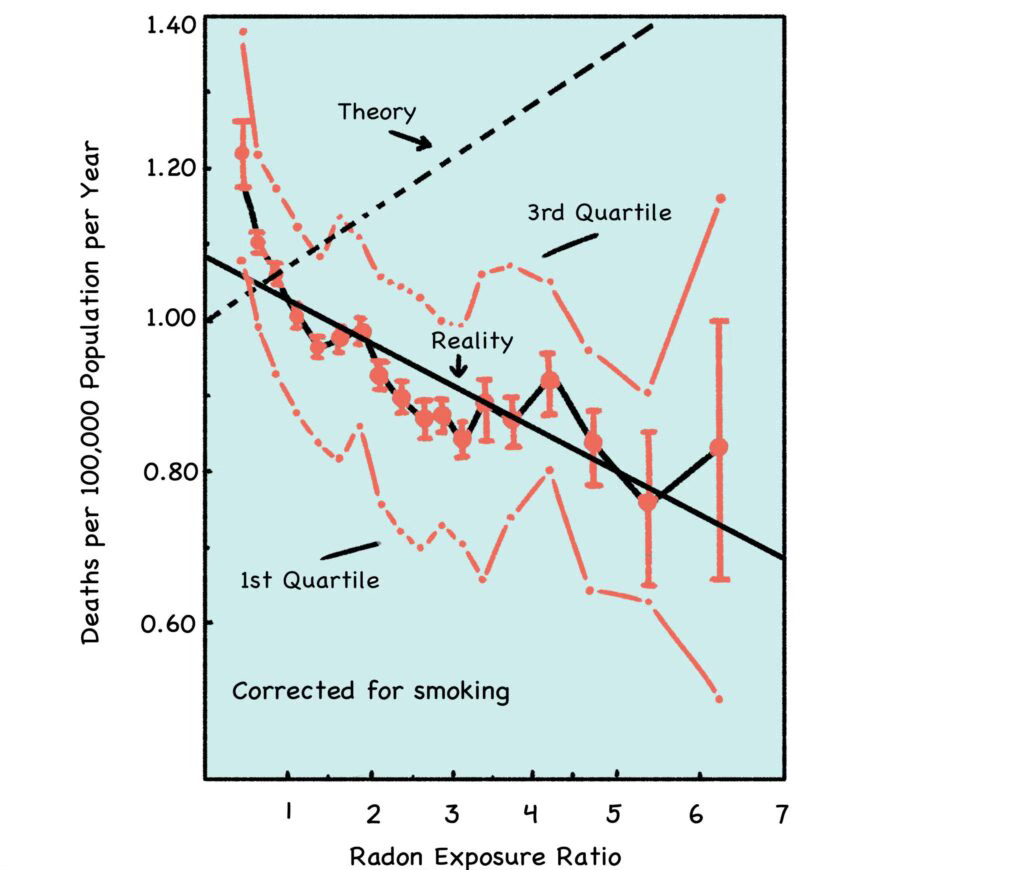
Fig. 7: Radon and Lung Cancer
Source: http://www.phyast.pitt.edu/~blc/LNT-1995.PDF
The x-axis shows radon exposure, and the y-axis shows lung cancer mortality. The dashed “Theory” line shows the predicted increase of radon-induced cancer per the LNT model. The solid line shows real-world cancer rates trending lower with increasing levels of radon.
2.1 SOME THINGS ARE MORE ZOOMACEOUS THAN OTHERS
On modernday planet Earth, the average background dose is about 3 mSv (milliSieverts) per year. But not to worry—our skin is a protective outer organ that shields our innards from most of the radiation we encounter. This is why the primary concern with nuclear material is inhaling or ingesting the stuff, rather than simply being around it. [6] This also explains why we should seal any openings to avoid inhaling fallout particles after a nuclear incident.
The overall biologic effect depends on the type of radiation, and the circumstances of contact:
- What is the collective energy of the zoomies that strike?
- Is the radiation source inside the body, or near the body?
- Is it partially blocked, slowed, or dispersed, or is it penetrating?
- If it penetrates, does it come in like a bowling ball, a BB, or a bullet (alpha, beta, or gamma ray)?
- What organ or organs are being struck?
- What is the intensity and duration of the zoomie storm (the dose-rate)?
All these variables must be considered when assessing a zoomie’s biologic effect, which is properly expressed in Sieverts. That’s because the focus of radiation safety is on the biologic effect of a received dose, typically called the effective dose. This is why Sievert is the preferred unit of measurement, rather than Gray, which measures the absorbed dose.
NERD NOTE: To be precise, one Sv represents the biologic effect that one joule of radiative energy has on one kilogram of living matter. Below 100 mSv, the distinction between Grays and Sieverts is often disregarded, and the terms are used interchangeably. (See Fig. 11 below. Also see our supplement “Sieverts and Grays.” [7]) Sometimes, however, these terms are not interchangeable. A good example is the Sievert measurement of an alpha particle’s effect. Because of the alpha’s +2 formal charge (which makes it very electron-hungry), and because of its size, the alpha particle is “weighted” on the Sievert scale as having twenty times the biologic effect of a stream of beta particles with the same collective kinetic energy.
Billions of years ago, when life on Earth was just getting started, background radiation levels were much higher than they are today. But as the eons passed, radioactive elements decayed, reducing background radiation. This happened because any radioactive atom is a decaying atom, throwing off bits and pieces as it transmutes into a series of other elements (a decay chain), until it finally stabilizes.
Most uranium eventually decays into lead. And not radioactive lead, just plain old boring lead, which is the freaky thing about radioactive material—it changes into entirely different stuff. Iron, for example, is always iron, no matter what: Rust is iron plus oxygen, but the iron is still there. But lead is not uranium plus anything. Iron doesn’t reduce to anything less than iron, while radionuclides (radioactive isotopes) will decay into completely different and lighter elements.
The alchemists were onto something; they were just fiddling with the wrong stuff. Radioactive decay, in which zoomies are released, is part of the ongoing process of transmutation, in which one substance, slowly or quickly, becomes an entirely different substance. It’s a simple sci-fi plot twist from there to conjure scare stories of mad scientists wielding death rays—the same pulp fiction / nuclear-fear button from a century ago that is still being pushed today.
Some decay chains are super-fast, lasting less than a second, and others can take forever. The uranium, thorium, and potassium in the earth’s crust, for example, have been decaying since the earth formed, more than four billion years ago.
In fact, more than half the heat in the earth’s core comes from the naturally-occurring radioactive decay of thorium, uranium, and potassium-40, along with the heat of friction from the circulating molten core, and a bit of leftover heat from the formation of the planet itself. [8] The thermal energy from this geodynamo keeps the earth’s outer core in a molten, or liquid, state, and radiation is responsible for most of it. That’s why Earth is a nuclear planet. [9]
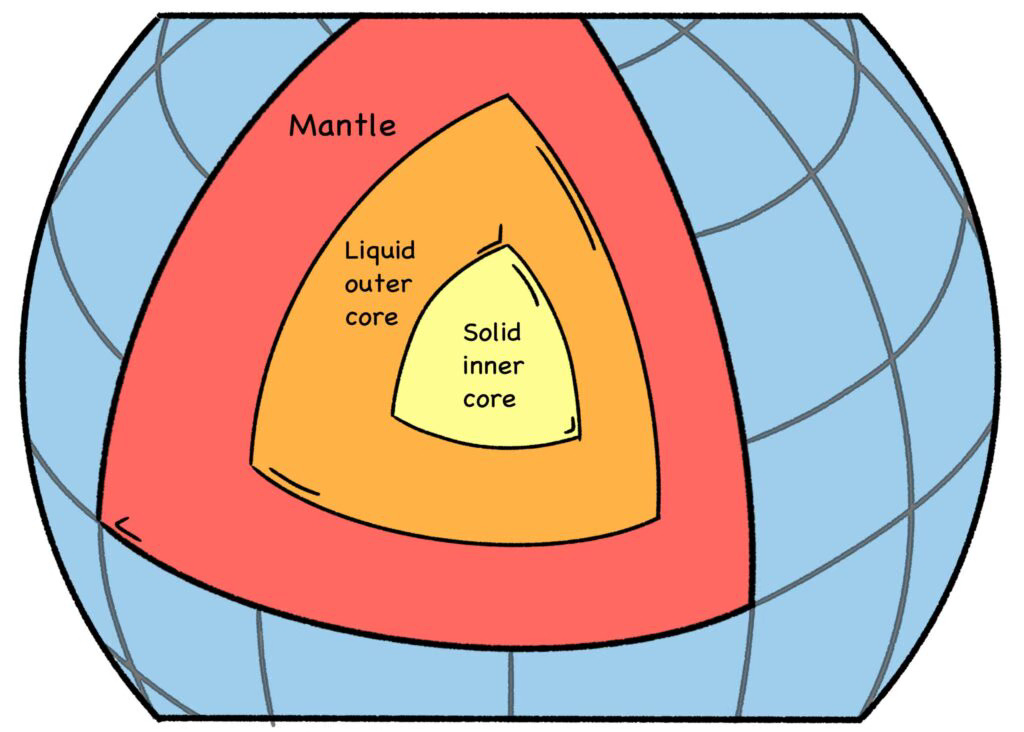
Fig. 8: Earth’s Core
The constant motion of the free charge within this molten metal, which is mostly iron, produces our planet’s magnetic shield. The magnetic shield is what deflects the charged particles of the solar wind (mostly high-energy protons), which would otherwise strip away Earth’s atmosphere.
Good planets are hard to find. NASA has recently discovered that over eons of time, the thin mantle of Mars allowed enough heat to escape, to where its core ceased being molten and thus stopped circulating. As a result, the red planet has no magnetic shield, and though the solar wind is much weaker that far from the sun, the rate of solar flux is still strong enough to strip away atmospheric gases. While some think it’s possible to create a Martian atmosphere for human colonization, others contend that without a magnetic shield, it would be an endless and Sisyphean effort to exceed the strip rate. [10]
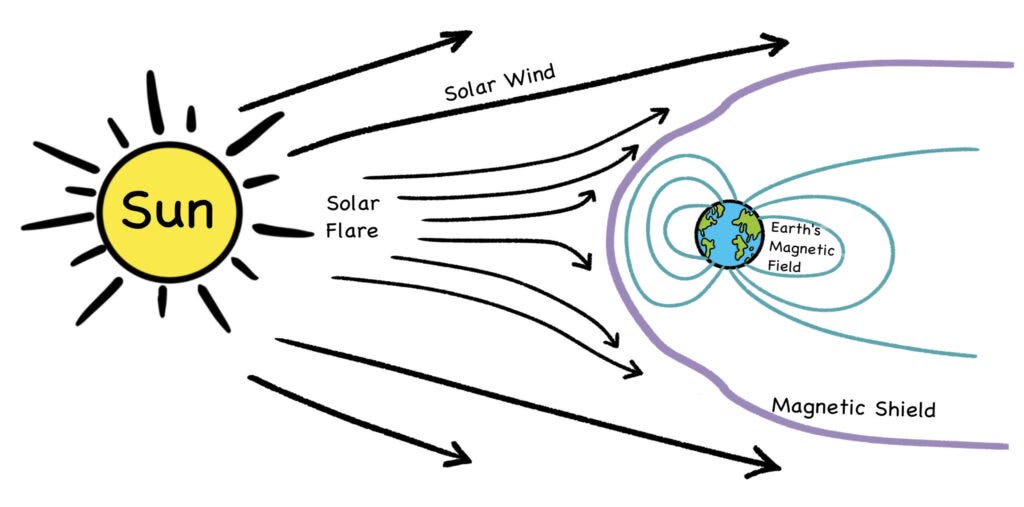
Fig. 9: Solar wind and magnetic shield
Whatever misgivings you may have about nuclear material, realize that this is indeed a nuclear planet—there wouldn’t be any life on Earth if it wasn’t. To take care of our home planet, we need to understand what makes it tick. And rather than fearing the very thing that makes life on Earth possible, we should use it to our best advantage. Everyone should have a healthy respect for radiation, but nuclear fear doesn’t help anyone at all (except the fossil fuel industry).
2.2 WHEN ZOOMIES STRIKE!
Toss a bullet at a wall and it bounces right off. Fire it from a gun and note the difference. In much the same way, a zoomie’s biologic effect is determined by its mass, speed, size, and electric charge. The predominating feature will depend upon the type of zoomies involved—protons, neutrons, and electrons, as well as the bizarre, positively-charged “anti-electrons” that decaying atoms sometimes emit, called positrons. (Gammas are different; we’ll explain below.)
ZOOMIES! WE’RE DOOMED!
An alpha particle can be stopped by a piece of paper or human skin, which has severa protective layers of dead cells that can stop alphas cold. So can a mere quarter-inch of air. But if a large dose of alpha particles is inhaled, their presence beside living lung tissue can pose a problem. This is why fallout dust can be a concern. However, ingested alpha emitters pass through the GI tract rather quickly.
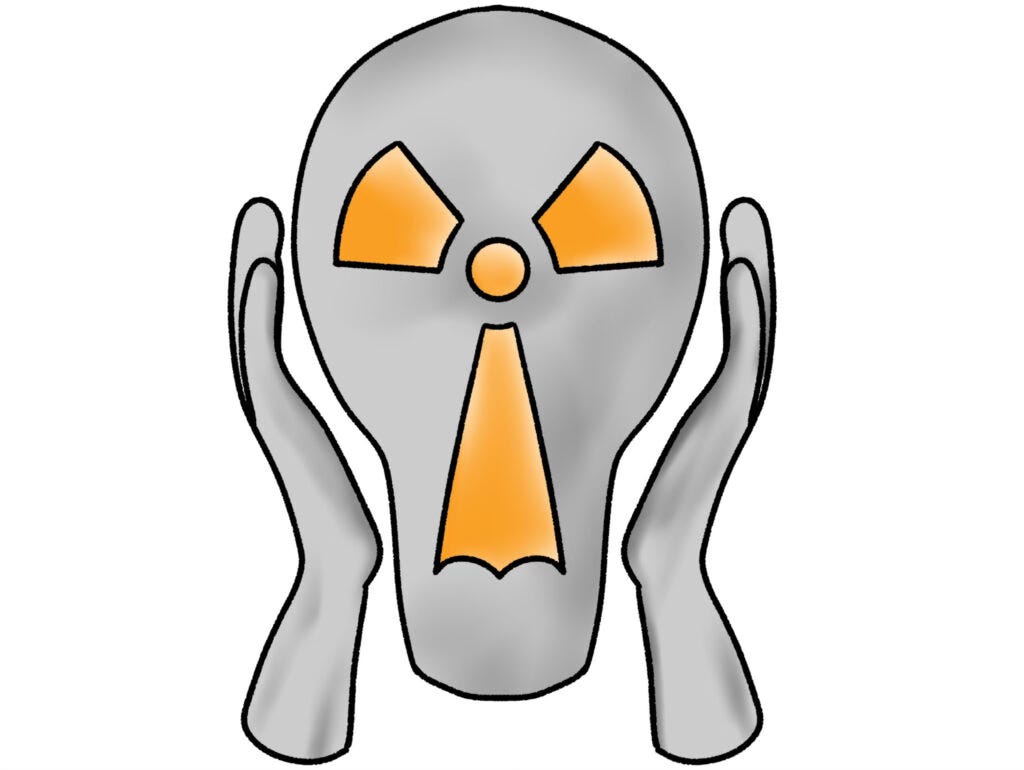
(Pg. 25) Credit: Scott E. Sutton
An alpha particle is essentially a helium nucleus—a tight bundle of two protons and two neutrons—but without helium’s two orbiting electrons. Since neutrons are electrically neutral (hence the term), the alpha’s two protons give it a formal charge of +2. This makes alpha particles electron-hungry.
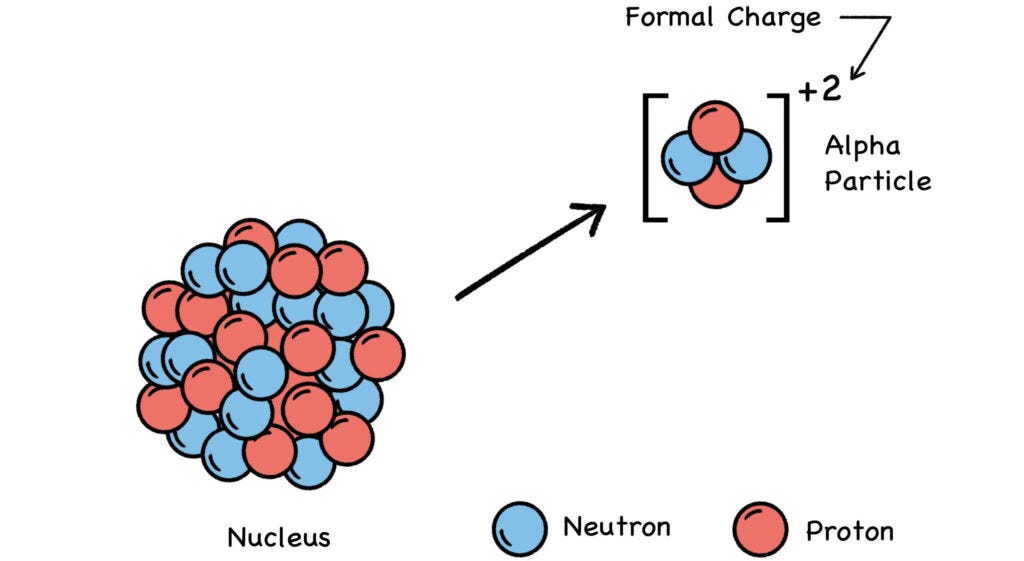
Fig. 10 Alpha Decay
The sheer size of an alpha particle—a cluster of four bowling balls in a world of penny-sized zoomies—is another reason why radiation’ biological effects are measure in Sieverts rather than Grays. Measuring radiation in Grays focuses solely on the amount of absorbed energy, and not on the type of particle, the size of the particle, or the biological effect of its impact.
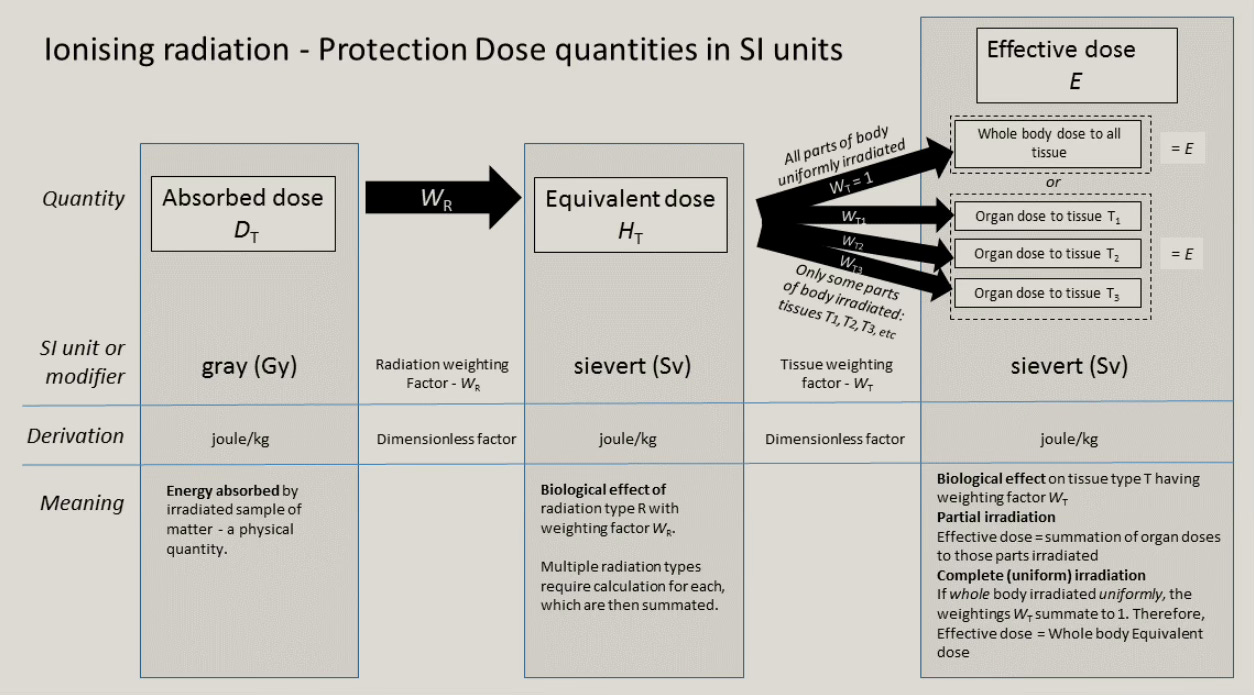
Fig. 11: Equivalency of Sieverts and Grays at Low Doses
Credit: By Doug Sim. Own work (CC-BY-SA-3.0)
Aside from its size and speed, the alpha’s biological effect is mainly due to its charge imbalance. After smashing through a cell membrane, the electron-hungry alpha will steal two electrons from the other atoms present, ionizing those atoms in the process. Hence the term ionizing radiation.
The alpha decay of radioactive material in the earth’s crust (i.e., the high-speed ejection of a helium-nucleus equivalent) is the world’s major source of helium: These alpha zoomies steal two electrons from whatever they slam into, and settle down to become helium atoms.
Elemental helium is a byproduct of the fossil fuel industry, a lightweight gas that comes to the surface along with heavier natural gas, crude oil, and coal. As the world weans itself from fossil fuel, our helium supplies will dwindle as well. And we’ve been squandering this limited resource filling up party balloons.
A beta particle comes in two flavors: negatively-charged electrons and their positively-charged equivalents called anti-electrons, or positrons. Betas are about 8,000 times smaller than alphas—just one tiny electron, compared to a massive cluster of two neutrons and two protons, which are nearly identical in size and mass.
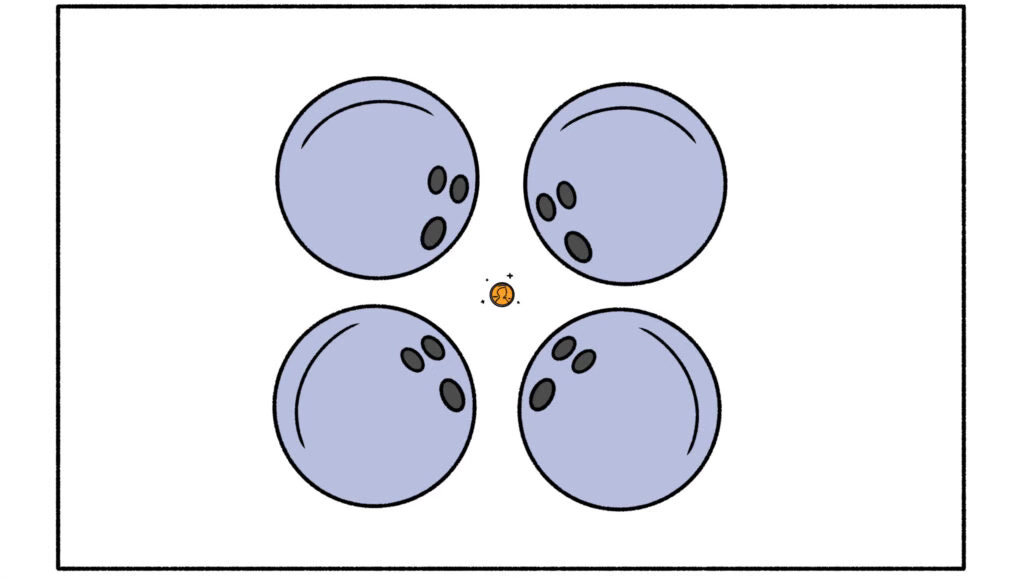
Fig. 12: Alpha Particle vs. Beta Particle
Like a penny vs. four bowling balls
While alphas can be stopped by a piece of paper, the tiny beta particle can be far more penetrating, depending of course on its energy level. When you’re that small, the solid wall of atoms that forms a piece of paper can be seen for what it actually is—mostly empty space. Nevertheless, betas can be stopped by a few millimeters of wood or aluminum, as they eventually hit something and fizzle out.
Gamma rays are high-frequency electromagnetic waves, but they can also act like “massless particles.” Due to the short wavelengths of their electric and magnetic fields, gammas are the most difficult type of radiation to block, and may have a substantial biologic effect depending on their energy level. These waves / packets of light can bestopped, but it requires a foot of lead, six feet of concrete, or about fifteen feet of water.
A gamma ray is a highly penetrating form of electromagnetic radiation, and can be thought of as a packet of pure energy—a photon, or discrete quantity of light. Depending on their total energy, gamma rays can impart a greater biologic effect than alphas or betas.
Like any form of electromagnetic radiation, light is very weird stuff—sometimes it acts like a particle, and sometimes it acts like a wave. This wave / particle duality is a fundamental aspect of the theory of quantum mechanics. [11]
At any energy level, gammas are simply “particles of light,” which can seem like a contradiction in terms if you’re not a quantum mechanic. [12] While photons have no “rest mass,” they do have “mass equivalence” as a result of their velocity, commonly denoted as C, or the speed of light. This is the equivalence of mass and energy that Einstein referenced in his formula E = mc².
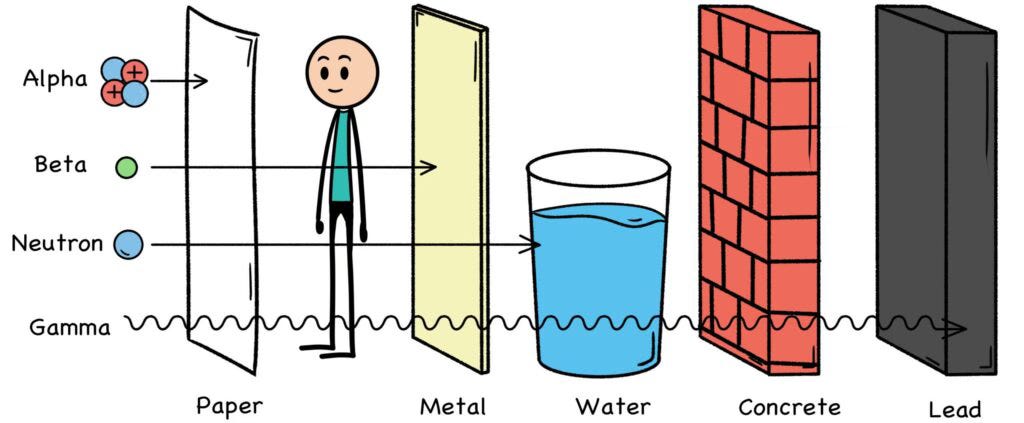
Fig. 13: Penetration of Various Zoomies
A zoomie can act like a cotton ball, a bowling ball, or a bullet, depending on its size, charge, and kinetic energy. At lower energies, a zoomie may only excite an atom, causing one of its electrons to temporarily jump to a higher orbital.
If a zoomie is energetic enough, it can knock an electron away from its home atom for good, turning the bereft atom, shorn of its electron, into an ion of its former self. Because of their charge imbalance, these ions (positive or negative) can either be more chemically stable or more chemically reactive than the atoms from which they originate.
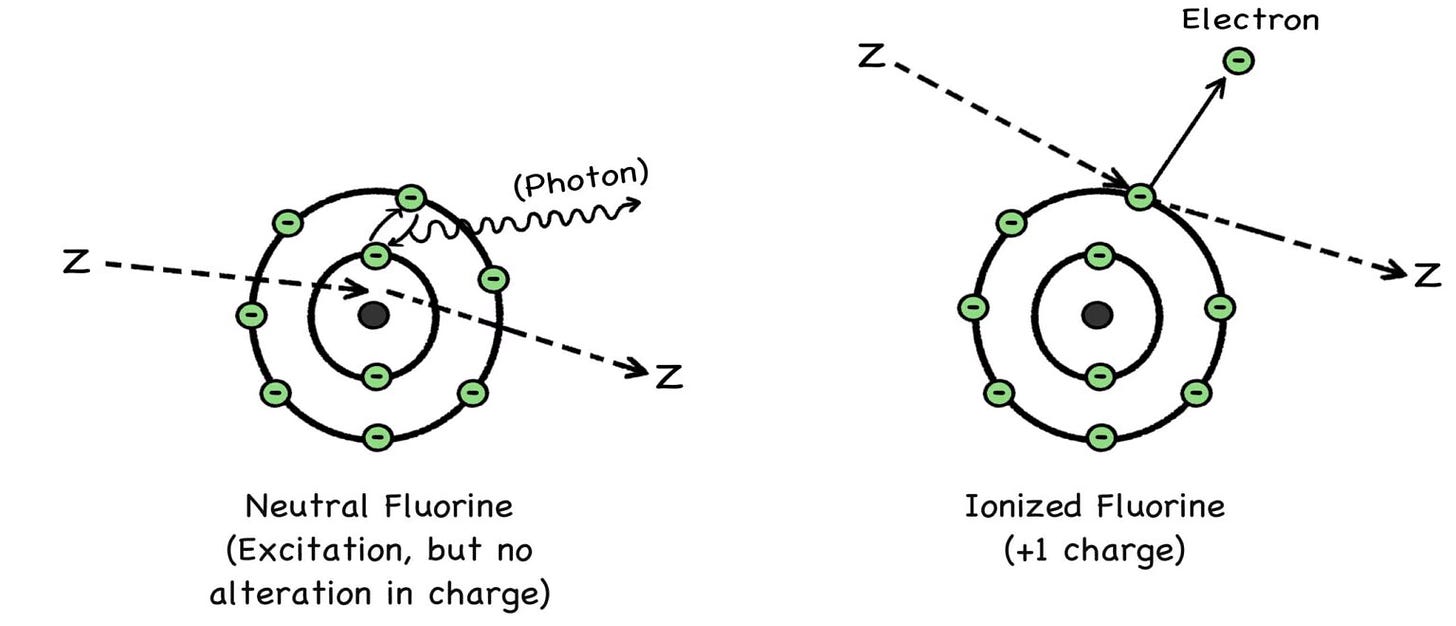
Fig. 14: Excitation vs. Ionization
So what’s the big deal? It’s just one atom, right? Maybe yes, and maybe no. Changing an atom’s ionic state can change the function of the molecule that atom is a part of. And if the molecule is in a strand of DNA, it must be repaired.
2.3 NO WORRIES
Living cells can perform up to ten thousand repairs per day, per cell. This is an underreported and pretty damn remarkable feat that we humans (and other living things) routinely accomplish every day of our lives. That deserves repeating:
Living cells can repair their own radiation and chemical damage, up to 10,000 times a day.
Science didn’t understand this in 1946, when the erroneous idea that “all radiation is harmful” gained an undeserved reputation as settled science. This false no-threshold claim persists to this day, inspiring a fresh outbreak of nuclear fear whenever a zoomie is found in the wild.
Research on cell repair began back in the 1930s. In 1951 Rosalind Franklin, a British chemist and X-ray crystallographer, discovered key aspects of the DNA molecule, recording her work in a series of X-ray photographs. [13] Without her
knowledge, one of her unpublished photos was shown to Watson and Crick by Franklin’s lab partner. From there, the three men detailed the structure of DNA’s double helix configuration, for which they, but not Ms. Franklin, received the Nobel Prize.
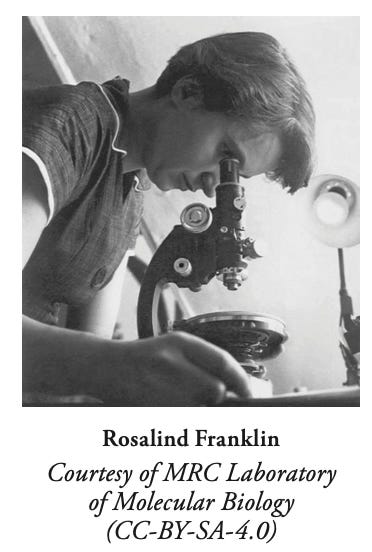
Understanding DNA structure greatly improved our ability to examine the hypothesis of cell repair. At the time, however, the very idea of cells repairing their own damaged DNA was dismissed by the biological sciences community at large. But research in the field doggedly progressed, so that “by the end of the 1970s, it was evident that cells have evolved multiple diverse mechanisms [of DNA repair].” [14]
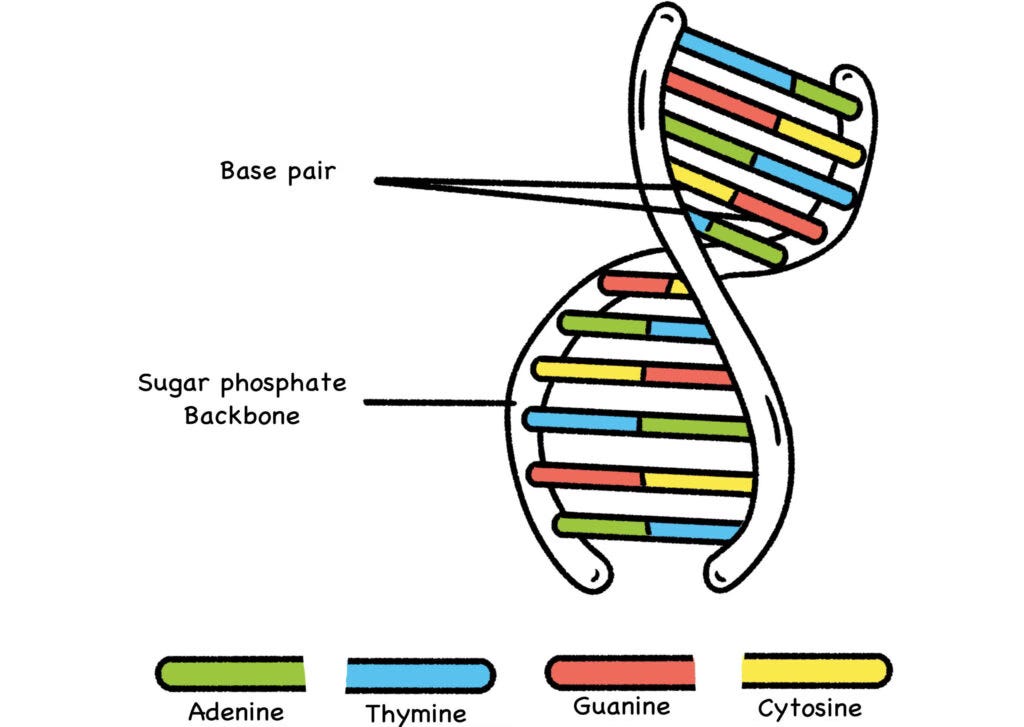
Fig. 15: Basic DNA Structure
Evidence continued to accumulate, and in 2015 the Nobel Prize in Chemistry was awarded to three of the principal pioneers in the now-established field of cell repair. [15] We humans host about ten trillion cells each, doing their regular jobs while also seeing to their own daily repairs. No wonder we need a good night’s sleep.
2.4 WAY, WAY BACK IN THE DAY
Uranium is element 92 on the Periodic Table. Most of the uranium found in nature is the U-238 isotope, with 92 protons and 146 neutrons. In any atom, the number of protons determines the element, while the number of neutrons determines the isotope. In the Periodic Table of Elements, the atomic number is the proton count, while the bottom number is the element’s average mass. Since many elements have multiple isotopes, the mass number is usually a weighted average.
Starting with sodium, element 11 on the Periodic Table, larger atoms tend to have more and more neutrons than protons. Down along the bottom of the Table, the actinides have more than half again as many neutrons as protons. The reason why is a bit complicated, but in simple-ish terms:
NERD NOTE: Since protons are positively charged, they naturally push each other away. This is the electrostatic force we’re all familiar with: Like charges repel and opposite charges attract.
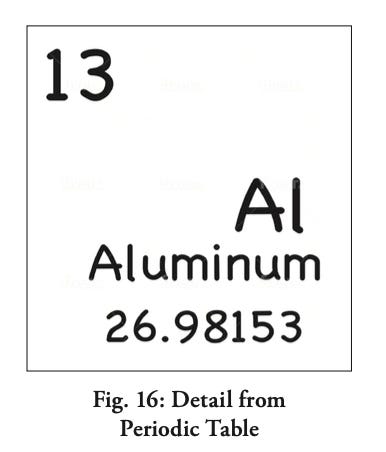
However, there is another force working at the subatomic level that most people are not familiar with:the strong nuclear force. This potent force makes positively-charged protons override their natural repulsion and attract each other instead, but the strong force is only effective across a very short distance.
Imagine forcing two magnets together so firmly that they’re just about to touch, and then they suddenly bind together. (Sub-atomic physics is weird; just roll with it.) Even though large clumps of protons will bind like this, each proton in the bunch will also repulse the protons they’re not directly bound to. Imagine a mosh pit of ravers in a group hug, even as they elbow the others around them—a tribal dance for Tasmanian Devils.
This is where neutrons come into play: As an uncharged, neutral version of a proton, neutrons can schmooze equally well with protons and each other. They serve as chaperones, the social diplomats that hold the mosh pit together and keep the party going, but it’s a trick that works less and less well in larger nuclei.
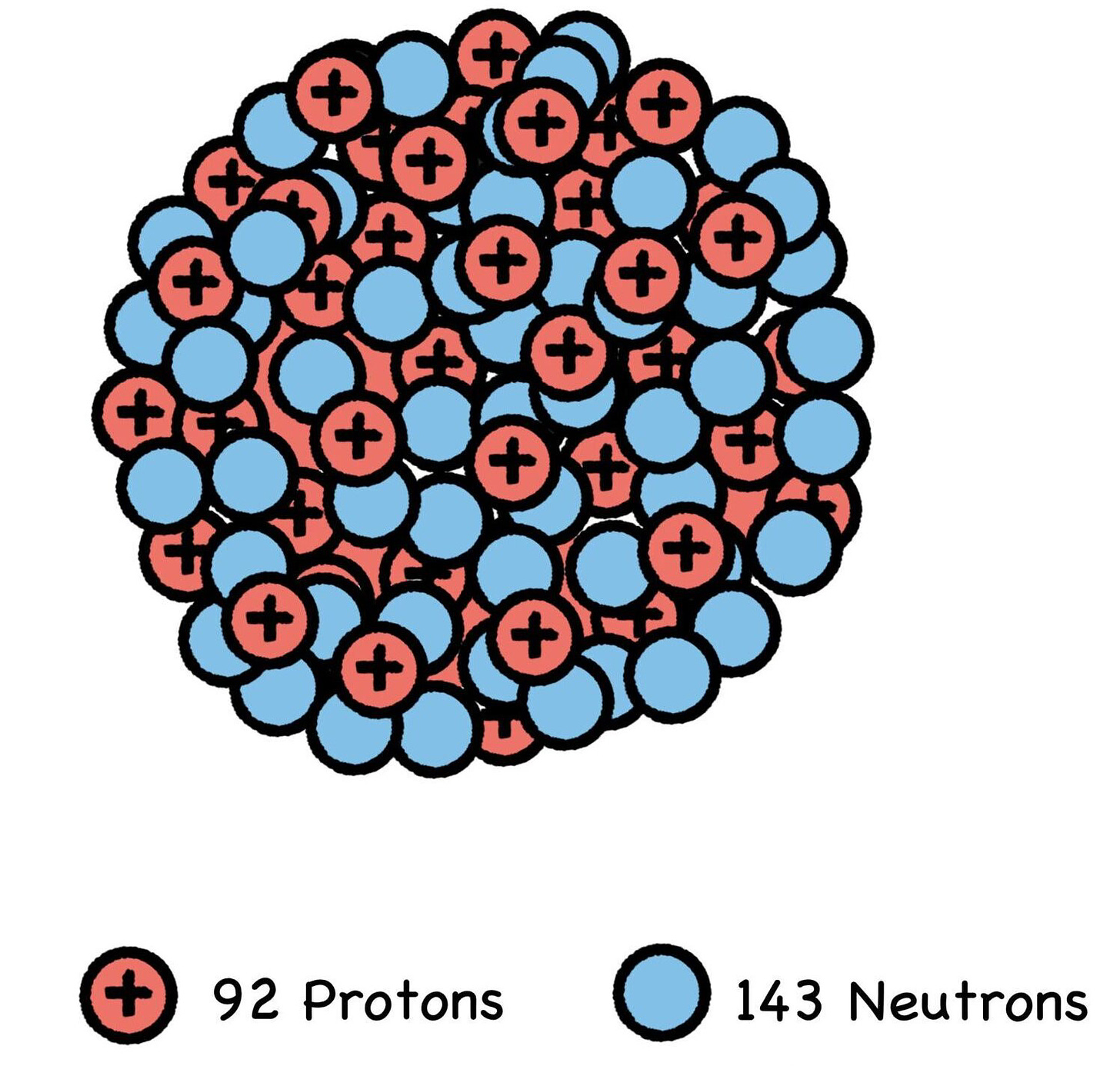
Fig. 17: Neutrons Help Hold the Nucleus Together
If we smack into one of these big, cobbled-together nuclei with a free-flying neutron zooming along at just the right speed, we can fission (split) the atom. This releases some of the fantastically compact and potent binding energy that’s been (barely) holding the nucleus together all these eons, along with the release of one, two, or sometimes three zooming neutrons. These fly off and split other fuel atoms, and thus we have chain reactions.
This is entirely unprecedented in the field of energy production. Prior to fission, energy typically came from burning various materials to release their chemical bonds or form new ones. At the atomic level, combustion rearranges fuel molecules into different molecules, but that’s about it. A lot of electrons get shuffled around, throwing off some energy in the process, but the nuclei of the atoms involved remain serenely undisturbed. Chemistry is basically electron management.
Splitting the atom is an entirely different process. And of all the atoms found in the natural world, the easiest one to split is uranium-235. Billions of years ago, natural uranium contained more than 5% U-235—a lighter, less stable, and thus more radioactive isotope of uranium. With the requisite 92 protons (that’s what makes it uranium) but a lower neutron count of 143, U-235 is like a stripped-down hot rod of the far more sedate uranium-238, the full-size atomic sedan.
With fewer neutrons to hold it together, a U-235 nucleus is easier to split with a free-flying neutron, which its neighboring U-235 atoms will sometimes spontaneously eject. This being the case, most of Earth’s unstable U-235 has long since decayed away or fissioned, leaving a concentration of just 0.7% in today’s natural uranium.
Way back in the day, the more radioactive U-235 isotope was everywhere, to one degree or another, in every Precambrian rock and puddle, and life had to adapt. Along with cosmic rays, uranium and some of the isotopes into which it decays were among the first evolutionary incentives, by stimulating the cell’s ability to repair its own radiation damage. This mechanism became an integral part of every living cell’s tool kit. If it didn’t, we wouldn’t even be here. That’s worth repeating:
The ability to repair radiation damage is part of every living cell’s tool kit.
The ability to self-repair radiation damage is the same mechanism our cells employ to handle the effects of chemical damage, such as oxidative stress. We take antioxidants to aid our metabolism, but most of us aren’t aware that our cells’ ability to repair oxidation damage is actually an adaptation of the earlier mechanism of radiation repair.
Similarly, most people aren’t aware that atmospheric oxygen (O₂) was an environmental latecomer, a corrosive substance that early life adapted to and learned to utilize. [16] Cells evolved to use the oxygen molecule, repairing the damage it caused by replicating their earlier success with radiation repair. The fact is, living cells had successfully adapted to radiation long before complex lifeforms drew their first collective breath. [17]
Even now, billions of years later, and despite how highly evolved we are, the oxygen we need to survive is still corrosive to our cells. As Reuter, et al., explain, “observations to date suggest that oxidative stress, chronic inflammation, and cancer are closely linked.” [18]
2.5 WELL, MAYBE SOME WORRIES
As rugged as cells have become in adapting to life on this nuclear planet, they can only do so much. If their rate of repair is overwhelmed by a zoomie storm, there will be trouble unless there is enough quiet time for repairs. But sometimes the zoomies come so hard and fast, and the storm lasts so long, that cells simply can’t recover. And whenthey die, flushing their carcasses out of your carcass can make you sick—hence the term “radiation sickness.” [19]
If the overirradiated cells don’t die, things can become even more problematic—their DNA may have been damaged to where they start making mutated copies of themselves. In concert with other factors, cell mutation can prompt a beneficial evolutionary adaptation, or it can lead to cancer and other maladies. But except for very high doses in the range of several Sieverts (an extremely rare occurrence), ionizing radiation is a weak cancer agent compared to most other natural hazards, such as the air we breathe and the food we eat. [20]
As scary as radiation has been made out to be—a sort of “sub- atomic Ebola”—the descendants of the Hiroshima and Nagasaki victims have shown no signs of inherited mutations, even though their forebears were irradiated more than any population in human history. [21] That’s also worth repeating:
The descendants of Hiroshima and Nagasaki victims have shown no signs
of inherited mutations.
Contrary to popular belief, the cancer rates among the hibakusha (the Japanese bomb survivors) are nearly identical to the wider Japanese population, differing by less than 1%. [22] This obviously does not justify the use of nuclear weapons, nor does it minimize their horrific effects. But of all the cancer risks we face, it does demonstrate just how ineffective radiation actually is.
It doesn’t matter where zoomies come from—kitty litter, guacamole, radon gas, bananas or beer, an X-ray or a CT scan, a nuclear reactor or outer space. The origin is irrelevant; the paramount concern is their health effect on humans and other living things. There are only three important details about any form of radiation:
• The dose, or cumulative energy, these subatomic particles and waves of photons impart as they strike, pass through, or pass close by a target (such as yourself)
• The rate, or the amount of time in which the dose is received
• The size of the affected area.
Zoomies are zoomies, whether they come from beyond the stars or a humble rock in the desert. If a zoomie plows through the periphery of a cell, it may not be a big deal. Even if it strikes a DNA strand, it might not be a big deal, either—unless that cell is in the process of dividing. This is because cells can’t always fully repair themselves during cell division (mitosis) or reproduction (conception). They can, however, repair themselves afterwards, but the process isn’t always foolproof.
This is why some researchers consider embryos and fetuses to be more susceptible to radiation damage—they’re constantly growing, meaning more cell divisions per unit of time. [23] However, since infants have stronger immune systems, others consider the net effect to be a wash.
This is also why radiation therapy can kill a patient’s hair, along with their targeted cancer. Since both hair and cancer cells have relatively rapid growth rates, they divide more often than other cells. This means there is a greater chance of zoomies hitting them during cell division, when they can’t repair the hit as easily. Thus, the faster cells grow, the more susceptible they are to radiation damage, with more opportunities to flub the “proofreading” they use to self-repair any damaged DNA. [24]
This also explains why radiation therapy includes a recovery period between sessions. A radiologist will take care to divide a dose into multiple beams converging on the target tissue. That way, the cells surrounding the target will receive a lower dose. Even so, your body needs time to repair the zoomie damage and flush out the dead cells.
2.6 A SPECIAL KIND OF AWFUL
Nuclear skeptics seem to think that radiation from the nuclear industry is somehow more harmful than natural background radiation, as if it possessed a special kind of awful, like an engineered toxin that doesn’t appear in nature.
Not true. Once again: Zoomies are zoomies. Like any other projectile, zoomies can be no big deal, or kind of a big deal, or a very big deal, indeed: cotton balls, BBs, bullets, or photon torpedoes. It all depends, because the issue is not just about dose, but dose-rate: how energetic the zoomies are (dose), and how many of them strike over what period of time (rate). Getting hit with a thousand cotton balls all at once would probably be unpleasant. But that doesn’t mean that getting hit with one per second, until you accumulate a total dose of one thousand hits, is unsafe. Annoying perhaps, but not unsafe.
So the next time you read an alarming article about “37 Trillion Becquerels!” see if the reporter mentions the two most important details of any radiation event—the dose (cumulative energy) and the rate (period of exposure), expressed together as the dose-rate. If they don’t mention these important factoids, they’re probably just trying to scare you with big numbers, or they themselves are scared and are spreading their nuclear fear like a virus. You can mask up by knowing the facts.
Background radiation is everywhere on this nuclear planet. But as things turned out, living cells (including ours) learned how to repair themselves, and we can all breathe easy because of this singular evolutionary development. Once again, we wouldn’t even exist if our cells lacked the ability to self-repair radiation damage. Even so, radiation is something that far too many people fear, regardless of the dose.
Like any kind of fear, nuclear fear can be highly contagious. The remedy, as always, is a generous serving of facts, seasoned with a bit of perspective. [25] But even more important than getting the facts straight is a person’s willingness to change their mind in light of new information. Few people have said it better than Bertrand Russell:
“The essence of the liberal outlook lies not in what opinions are held, but in how they are held: instead of being held dogmatically, they are held tentatively, and with a consciousness that new evidence may at any moment lead to their abandonment.”